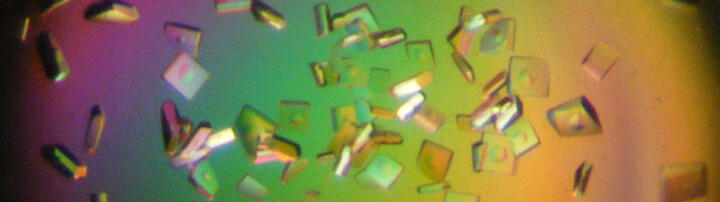
Daumke Lab
Structural Biology of Membrane-Associated Processes
Structural insights and cellular function of EHD ATPases
Eps15 homology domain containing proteins (EHDs) are ubiquitously expressed dynamin-related ATPases which are built of an amino-terminal GTPase domain, a central helical domain and a carboxy-terminal regulatory Eps15 homology (EH) domain. The four mammalian EHD members are found at the plasma membrane or at vesicular and tubular endocytic membrane structures. EHDs have been implicated in several membrane trafficking pathways, most notably during the recycling of receptor proteins back to the plasma membrane.
Figure 1: a) EHD2 oligomerization around negatively-charged liposomes inducing tubulation in ring-like oligomers. b) Crystal structure of the EHD2 dimer (pdb 2QPT) assembled via a conserved interface in the GTPase domain. c) Proposed oligomerization model of EHD2.
During my PostDoc time, I determined the crystal structure of the EHD2 dimer, which demonstrated that stable dimerization of EHD2 is mediated via the GTPase domains (Daumke et al., Nature, 2007) (Fig. 1). I furthermore showed that EHD2 oligomerizes in ring-like structures around tubulated liposomes.
We subsequently discovered that EHD2 localizes to the neck of caveolae leading to their stabilization in the plasma membrane (Moren et al., Mol Biol Cell, 2012). By a combination of X‑ray crystallography and electron paramagnetic spin resonance, we identified the primary membrane-binding sites of EHD2 at the tip of the helical domain (Shah et al., Structure, 2014). Also, the N-terminus of EHD2 interacts with membranes and may constitute a molecular switch that controls oligomerization.
In the EHD2 crystal structure, the two helical domains protrude in parallel, constituting the closed conformation of the dimer. In a follow-up study, we determined a second, open conformation of an EHD4 dimer in which the helical domains protrude away from each other (Melo et al., PNAS, 2017).
Figure 2: Structural insights into EHD4 assembly on membranes. a) Tomogram reconstruction of EHD4-covered membrane tubes. The boxed area is magnified in the left bottom corner. Scale bar: 100 nm. b) Projections of subtomogram averages of individual tubes with different diameters and different helical families. Cross section (top) and Z projections (bottom) are shown. c) Projections of the membrane-bound EHD4 map. d) Cryo-ET map (grey surface) of the asymmetric unit, including the fit model. e) Reconstructed EHD4 right-handed helical filaments wrapping around a membrane tube. Each filament is indicated in a different color.
More recently, we reconstituted EHD4 helical filaments on membrane tubes of different diameters and determined their structures by cryo-electron tomography (Melo et al., Nat Commun, 2022) (Fig. 2). Thereby, we delineated the assembly interfaces of EHDs. Oligomerized EHD4 resembles the closed conformation, where the tips of the helical domains protrude in parallel into the membrane. Notably, EHD4 filaments adapted to increasing membrane curvature by increasing their pitch. This could be explained by modelling EHD filaments as an elastic ribbon which allowed us to calculate the spontaneous filament curvature of approximately 1/70 nm-1. This relative high value indicates that EHD4 prefers binding to membranes of low curvature, consistent with its reported localization at early endosomes and macropinosomes. Combining the available structural and functional data, we presented a refined model of EHD-mediated membrane remodeling.
In a collaborative project, we characterized the physiological function of EHD2 by generating a knockout mouse model. We discovered increased lipid droplet size in fat tissue of mice devoid of EHD2 (Matthaeus et al., PNAS, 2020). On the cellular level, we observed increased fatty acid uptake via a caveolae-dependent pathway. Concomitantly, elevated numbers of detached caveolae were found in brown and white adipose tissue lacking EHD2, and increased caveolar dynamics in mouse embryonic fibroblasts. EHD2 expression was down-regulated in the visceral fat of two obese mouse models and obese patients. Our data suggest that EHD2 controls a cell-autonomous, caveolae-dependent fatty acid uptake pathway and imply that low EHD2 expression levels are linked to obesity.
We also investigated the role of EHD2 on endothelial nitric oxide synthase (eNOS)-dependent vessel relaxation (Matthaeus et al., PloS One, 2019). Similar as in fat tissue, loss of EHD2 in small arteries led to increased numbers of caveolae detached from the plasma membrane. Concomitantly, impaired relaxation of mesenteric arteries and reduced running wheel activity were observed in EHD2 knockout mice. EHD2 deletion or knockdown led to decreased production of nitric oxide (NO), and eNOS was redistributed from the plasma membrane to internalized detached caveolae in EHD2-lacking cells. Our data suggest that EHD2-controlled caveolar dynamics orchestrates the activity and regulation of eNOS/NO at the plasma membrane.
Publications
- Daumke, O., Lundmark, R., Vallis, Y., Martens, S., Butler, P.J., and McMahon, H.T. (2007). Architectural and mechanistic insights into an EHD ATPase involved in membrane remodelling. Nature 449, 923-927. https://doi.org/10.1038/nature06173.
- Moren, B., Shah, C., Howes, M.T., Schieber, N.L., McMahon, H.T., Parton, R.G., Daumke, O., and Lundmark, R. (2012). EHD2 regulates caveolar dynamics via ATP-driven targeting and oligomerization. Molecular biology of the cell 23, 1316-1329. https://doi.org/10.1091/mbc.E11-09-0787.
- Shah, C., Hegde, B.G., Moren, B., Behrmann, E., Mielke, T., Moenke, G., Spahn, C.M.T., Lundmark, R., Daumke, O., and Langen, R. (2014). Structural insights into membrane interaction and caveolar targeting of dynamin-like EHD2. Structure (London, England : 1993) 22, 409-420. https://doi.org/10.1016/j.str.2013.12.015.
- Melo, A.A., Hegde, B.G., Shah, C., Larsson, E., Isas, J.M., Kunz, S., Lundmark, R., Langen, R., and Daumke, O. (2017). Structural insights into the activation mechanism of dynamin-like EHD ATPases. Proceedings of the National Academy of Sciences of the United States of America 114, 5629-5634. https://doi.org/10.1073/pnas.1614075114.
- Melo, A.A., Sprink, T., Noel, J.K., Vázquez-Sarandeses, E., van Hoorn, C., Mohd, S., Loerke, J., Spahn, C.M.T., and Daumke, O. (2022). Cryo-electron tomography reveals structural insights into the membrane remodeling mode of dynamin-like EHD filaments. Nature communications 13, 7641. https://doi.org/10.1038/s41467-022-35164-x.
- Matthaeus, C., Lahmann, I., Kunz, S., Jonas, W., Melo, A.A., Lehmann, M., Larsson, E., Lundmark, R., Kern, M., Blüher, M., et al. (2020). EHD2-mediated restriction of caveolar dynamics regulates cellular fatty acid uptake. Proceedings of the National Academy of Sciences of the United States of America 117, 7471-7481. https://doi.org/10.1073/pnas.1918415117.
- Matthaeus, C., Lian, X., Kunz, S., Lehmann, M., Zhong, C., Bernert, C., Lahmann, I., Muller, D.N., Gollasch, M., and Daumke, O. (2019). eNOS-NO-induced small blood vessel relaxation requires EHD2-dependent caveolae stabilization. PloS one 14, e0223620. https://doi.org/10.1371/journal.pone.0223620.
Researchers in my group
Elena Vázquez Sarandeses (Doctoral student)
Jeff Noel (PostDoc 2015 – 2021)
Thiemo Sprink (PostDoc 2017 – 2020)
Claudia Matthäus (PostDoc 2016 – 2019)
Arthur Melo (Doctoral student/PostDoc 2013 – 2018)
Claudio Shah (Doctoral student 2008-2013)
Main collaborations
Richard Lundmark, University of Umeå
Harvey McMahon, LMB Cambridge
Ralf Langen, University of Southern California
Carmen Birchmeier, MDC
Maik Gollasch, ECRC Berlin
Press releases